Despite its promise of offering low-carbon energy at a stable generation rate, nuclear energy’s bad reputation precedes it in many countries. While overall public support in favor of using nuclear energy to combat climate change is high, more than a quarter of Europeans and Americans still opine against it. Despite the aftermath of disasters such as Chernobyl and Fukushima, nuclear power capacity is set to grow in the coming decades, especially in Asia.
The IEA’s, in its annual World Energy Outlook report, states an installed nuclear capacity growth of over 26% from 2020 to 2050 (reaching about 525 GWe). More importantly, it estimates that a significant investment in existing and new nuclear power plants is necessary for us to effectively transition away from coal and gas and maintain our emissions reduction trajectories. For this to happen, nuclear has to sort of, be reborn and seen in a new light from different perspectives.
Safety, hazardous waste concerns, building/ deployment time, and plant size are the main factors that hinder nuclear expansion. These factors and their solutions will be tackled in this article which unlike its name will focus on both fission as well as fusion-based technologies. Hope is, that a new generation of startups promise an upgraded type of reactor (and ancillary technologies). One that is smaller, cheaper, and faster to design; but is still very much safe, reliable, and easier to deploy anywhere than their predecessors.
1. Small Modular Reactors (SMR)
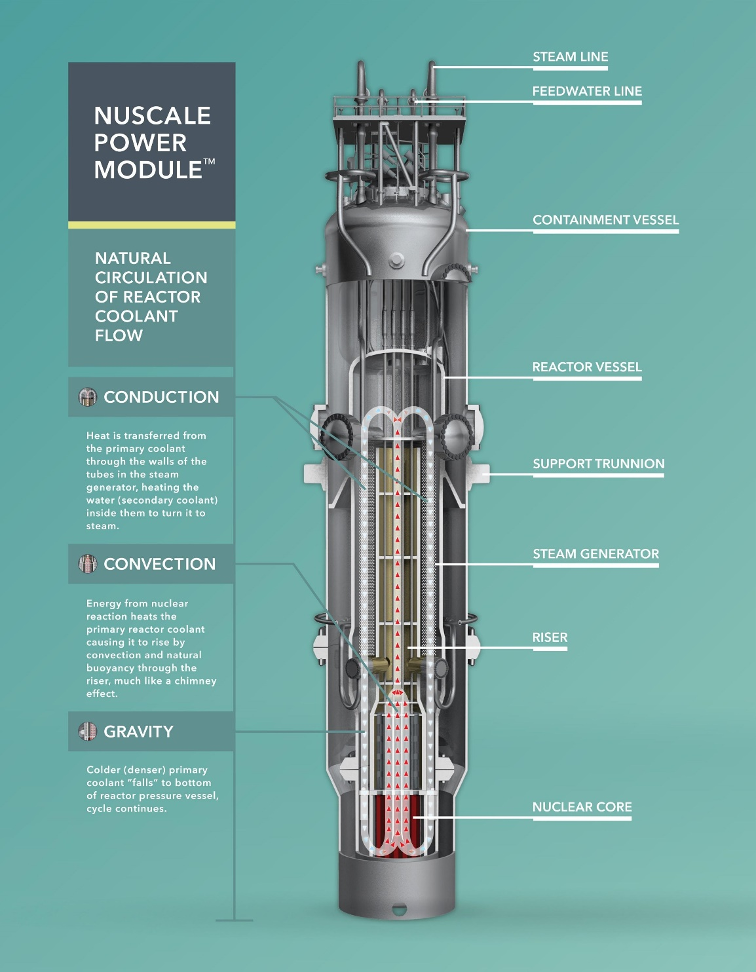
Small modular reactors (SMRs) are nuclear fission reactors that are smaller than conventional nuclear reactors and typically have an electrical power output of less than 300 MWe. Due to their compact size (< 50 tons), they can be transported around conveniently and can be installed anywhere on/ off-grid. Another aspect is scalability where reactors can be added together to amplify the power output manyfold. The idea here is for these SMRs to be flexible, and act as a “load-follow” to renewables. This keeps an electrical grid balanced as renewables like sun and wind rise and fall, and demand varies. Their modular nature implies that they are also less expensive. A major factor that separates an SMR from conventional nuclear power plants is that their cores are much smaller, and they have fewer moving parts. They also have safety features including automatic shut-off and indefinite cooling without the need for water.
2. Fully Ceramic Micro-Encapsulated (FCM) Fuel
It isn’t sufficient to make the reactor safe though. That’s where FCM fuel comes in. The idea is to coat the core fuel (uranium) kernel with multiple layers of fission products (TRISO particle fuel) and encase this within a fully dense silicon carbide (SiC) matrix instead of a graphite matrix that current TRISO-based fuels use. This ensures high mechanical and chemical stability during irradiation and temperature changes. The higher-thermal conductivity of FCM fuel makes it extremely rugged and stable across a wide range of temperatures, while the SiC prevents the escape of fission products even if a TRISO particle should rupture during operation.
3. Stable Salt Reactor (SSR)
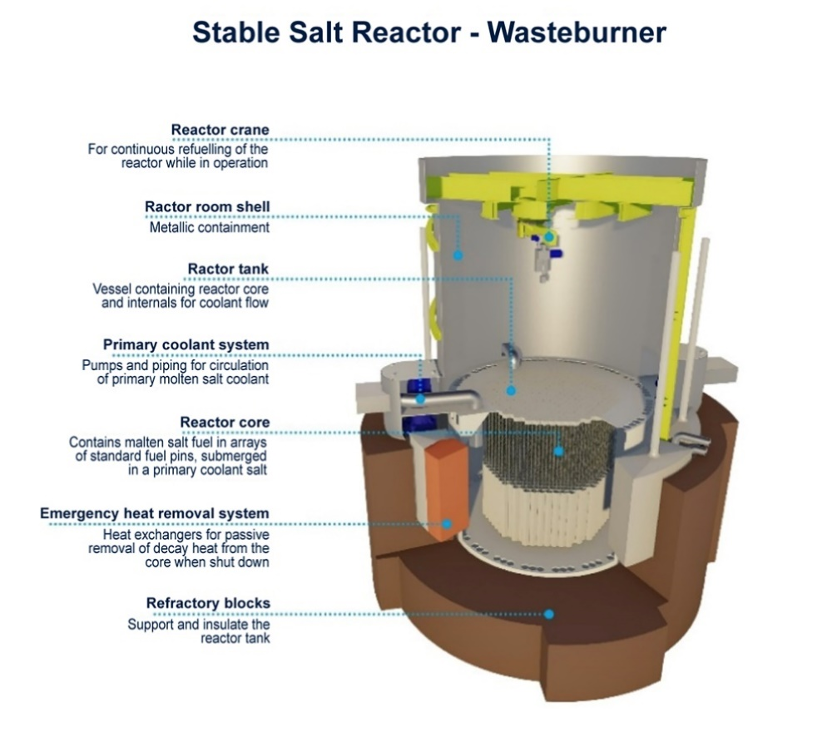
Designed and developed by Moltex Energy, the SSR is effectively an SMR that operates on nuclear waste. Using molten salt rather than water for fueling and cooling, allows radioactive gases to be absorbed by solidifying the salt in case of an emergency thus preventing a meltdown. The capital cost of the stable salt reactor is estimated at $1,950/kW. For comparison, the capital of large-scale nuclear is $5,500/kW.
4. The Microreactor
Built to be both reliable and economical, the portable microreactor developed by Radiant Nuclear offers a peek into the future of truly decentralized microgrids. Each one of these units can deliver over 1 MegaWatt of electricity and can operate for up to 8 years. They can fit into your average shipping container and provide enough power to support over 1,000 homes per unit. Due to its high portability and quick deployability, remote communities and disaster areas are key use cases for such reactors.
The Orbitron
Nuclear fusion is generally thought of as expensive and expansive; A fusion reactor needs large lasers or magnets to convert hydrogen to plasma under million-degree temperatures. Also, not to forget plenty of personnel. In sharp contrast, Avalanche Energy’s small-scale modular approach seems to mimic Tesla’s approach with batteries. It comprises of hundreds of little reactors called orbitrons; each of which employ electrostatic fields to trap fusion ions, while also employing a magnetron to reach higher ion densities. The resulting fusion reaction produces neutrons that can be transformed into heat. Measuring just approximately 5 inches in diameter (not larger than a fire extinguisher), a single orbitron can produce 5 to 15 kilowatts of power. Historically, a fusion experiment took years, if not decades, and billions of dollars to set up, but with the orbitron real-world experiments could be run a few times per week on single-digit millions of dollars.
All is not hunky dory though...
The major obstacle for SMRs comes in the form of radioactive waste. Due to their smaller size: power ratio, the SMR designs produce not less, but potentially much more waste: more than five times the spent fuel per unit of power, and as much as 35 times for other forms of waste. Other problems include neutron leakage which makes waste decommissioning much more precarious. Figuring out long-term storage and the potential recycling of spent nuclear fuel is of paramount importance for the future of SMR technology.
Parameter/ Reactor | Energy Density (MJ/kg of fuel) | Installation costs ($/kW) | Safety | Reliability | Waste Disposal |
---|---|---|---|---|---|
Conventional Coal | 30 | 3500-3800 | Average | Good | Average |
Conventional Nuclear (U235 fuel) | 79,390,000 | 5500-6000 | Average | Good | Below Average |
SMR | 1/10th of conventional nuclear | 2000-4000 | Good | Good | Below Average |
SSR-W (LiF-BeF salt) | 1/10th of conventional nuclear | 1950 | Good | Good | Below Average |
Conclusion
Although the sun itself is some 90 million miles away from us, we are getting quite good at mimicking this marvel of energy. The commercial nuclear (fusion) market is expected to boom in the upcoming years. The International Energy Agency (IEA) estimates that if $1 trillion of the $9.5 trillion in global renewables investment planned over the next 20 years, is invested in fusion projects, it could deliver electricity to the grid by as early as 2030. There are at least 35 companies working on fusion worldwide, and investments in these stood at more than $ 30 billion as of 2021. Small modular reactors will be an exciting next step in commoditizing energy for the common man. Good things do indeed come in small packages.
Leave A Comment